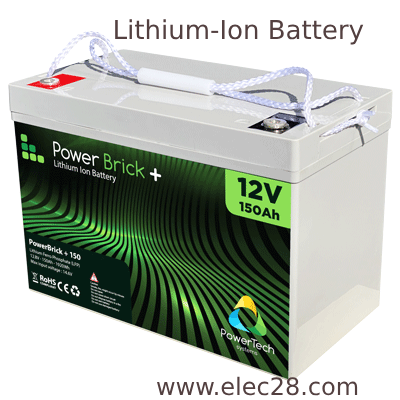
I. Introduction
A. Definition and a brief history of lithium-ion batteries
Lithium-ion batteries stand at the van of ultramodern energy storehouse results, revolutionizing colorful diligence with their remarkable parcels. Firstly developed in the 1970s, lithium-ion batteries have since become ubiquitous in consumer electronics, electric vehicles, and renewable energy systems. Their unequaled energy viscosity, featherlight design, and rechargeable nature have propelled them to elevation, powering the bias and technologies that define our diurnal lives.
B. Importance of lithium-ion batteries in ultramodern technology
Lithium-ion batteries represent a significant cornerstone in the elaboration of battery technology. Before their arrival, nickel-cadmium and lead-acid batteries dominated the request but were agonized by issues similar to low energy viscosity, limited cycle life, and environmental enterprises. The preface of lithium-ion batteries addressed these failings, steering in a new period of movable electronics, electric transportation, and grid-scale energy storehouses.
II. Chemistry of Lithium-Ion Batteries
A. Explanation of the components and chemical reactions involved
The complex chemical responses that take place inside the factors of lithium-ion batteries are what drive their operation. The anode, cathode, and electrolyte are the three primary corridors of these batteries in most cases. Lithium ions travel between these parts when the device is operating, which makes it easier for energy to be stored and for electrical current to flow.
B. Discussion on the anode (typically graphite), cathode (usually lithium metal oxides), and electrolyte
Graphite-based anodes, which are the core of lithium-ion batteries, are what power them. During intercalation, which occurs during battery charging, lithium ions are taken from the cathode and placed into the anode’s graphite structure. When the anode discharges, these lithium ions that have been stored there are free to participate in the electrochemical reactions that produce electrical energy. As preliminarily mentioned, lithium substance oxides, similar to lithium cobalt oxide( LiCoO2) or lithium iron phosphate( LiFePO4), frequently comprise the cathode of a lithium-ion battery. When the anode discharges, these lithium ions that have been stored there are free to share in the electrochemical responses that produce electrical energy. There, they are incorporated into the material’s essential oxide crystal structure. As a result of the reverse process, energy is released in the form of an electrical current when the lithium ions return to the anode during discharge.
The electrolyte moves lithium ions from the anode to the cathode. Lithium mariners are among the materials that are often liquid or gel-like when being dissolved in detergent. The electrolyte is necessary to facilitate ion mobility and prevent direct contact between the anode and cathode, which helps prevent short circuits. The intricate connections between the anode, cathode, and electrolyte in lithium-ion batteries allow for the storage and release of electrical energy. To maximize battery effectiveness, safety, and performance in instigative conditioning, it’s essential to comprehend these chemical relations.
III. Working Principle
A. Overview of the charging and discharging processes
The electrochemical energy storage concept, which lets lithium-ion batteries store and distribute electrical energy effectively, is a straightforward yet clever idea. Lithium ions are transported via the electrolyte, where they are stored, from the positive electrode (cathode) to the negative electrode (anode) while charged. Again, during discharge, the ions resettle back to the cathode, releasing energy that can be used to power colorful biases and systems.
B. Explanation of ion movement between the electrodes during operation
The movement of lithium ions between the electrodes is eased by the electrolyte, generally, a lithium swab dissolved in a detergent. When the battery is charged, lithium ions are uprooted from the cathode material( generally a lithium essence oxide) and resettle through the electrolyte to the anode( generally graphite). This process involves the reversible intercalation of lithium ions into the demitasse chassis of the anode material, where they’re stored until demanded for discharge.
Understanding the complications of ion movement within lithium-ion batteries is pivotal for optimizing their performance and life. The effectiveness of ion transport and intercalation processes directly impacts the battery’s energy viscosity, cycle life, and overall trustability. thus, ongoing exploration and development sweats concentrate on enhancing electrode accouterments, electrolyte phrasings, and cell design to ameliorate ion conductivity and minimize losses, eventually leading to more effective and durable lithium-ion battery systems.
IV. Advantages and Limitations
A. pressing the advantages of lithium-ion batteries, similar to high energy viscosity and long cycle life
Lithium-ion batteries offer a myriad of advantages that have propelled them to the van of energy storage technology. One of their primary strengths lies in their high energy viscosity, allowing for further energy to be stored in a lower and lighter package compared to other battery chemicals. This characteristic makes lithium-ion batteries ideal for movable electronics, electric vehicles, and other operations where space and weight are critical factors.
Also, lithium-ion batteries have an emotional cycle life, meaning they can suffer multitudinous charge-discharge cycles without significant declination in performance. This life makes them cost-effective over the long term, as they can endure times of use without demanding frequent relief. Comparing single-use batteries to lithium-ion batteries, which can be recharged and used repeatedly, results in less waste and less environmental effect.
B. Addressing limitations such as safety concerns and environmental impact
Still, lithium-ion batteries aren’t without their limitations and challenges. Safety enterprises have been a prominent issue, particularly in operations where batteries may be subordinated to extreme conditions or mechanical stress. Lithium-ion batteries are susceptible to thermal radiation and the threat of fire or explosion if inaptly handled or damaged. As a result, expansive exploration and development efforts are ongoing to enhance the safety features of lithium-ion batteries and alleviate these pitfalls.
Also, the environmental impact of lithium-ion batteries, particularly in terms of raw material birth, manufacturing processes, and end-of-life disposal, has raised concerns among enterprises about sustainability. Rudiments similar to lithium, cobalt, and nickel, which are crucial factors of lithium-ion batteries, are frequently sourced through mining operations with associated environmental and social counteraccusations. In addition, there are still gaps in the framework for lithium-ion battery recovery, which makes managing battery waste in the long run challenging.
In conclusion, lithium-ion batteries have downsides with regard to environmental sustainability and safety, indeed if they offer several benefits in terms of energy density, cycle life, and reusability. Lithium-ion batteries need to be delved and developed further to overcome these obstacles and guarantee that they’re a feasible and sustainable energy storage solution going forward.
V. Applications
A. Overview of various applications, including consumer electronics, electric vehicles, and renewable energy storage
Lithium-ion batteries find wide-ranging applications across different disciplines. They power consumer electronics like smartphones, laptops, and tablets, furnishing movable energy results. In the automotive sector, electric vehicles rely on lithium-ion batteries for propulsion, enabling cleaner and more sustainable transportation options. Likewise, lithium-ion batteries play a pivotal role in renewable energy storehouses, easing the integration of solar and wind power into the electrical grid. As renewable energy sources become more current, the demand for effective energy storage continues to rise. Lithium-ion batteries offer a dependable and scalable option for storing redundant energy generated from renewable sources, increasing their value during times of high demand or low generation.
B. Discussion on emerging applications and future trends
Looking ahead, rising operations similar to grid-scale energy storehouses, wearable technology, and medical bias hold a pledge to further expand the mileage of lithium-ion batteries. Continued exploration and development sweats aim to enhance battery performance, safety, and sustainability, paving the way for unborn inventions in energy storage and electrification.
VI. Conclusion
Lithium-ion batteries play a pivotal role in powering ultramodern technology, from smartphones to electric vehicles and renewable energy systems. Their significance lies in their versatility, offering high energy viscosity, rechargeability, and felicity for colorful operations. As technology advances, lithium-ion batteries will continue to drive invention and shape the future of energy storage.